Search for Articles
Decision making under virtual environment triggers more aggressive tactical solutions
Journal Of Digital Life.2023, 3,9;
Received:April 29, 2023 Revised:June 5, 2023 Accepted:June 7, 2023 Published:August 4, 2023
- Daisuke Murakawa
- Department of Health and Sports Sciences, Faculty of Health and Medical Science, Kyoto University of Advanced Science
- Shota Yamagata
- Faculty of Physical Education, National Institute of Fitness and Sports in Kanoya
- Yohei Takai
- Faculty of Physical Education, National Institute of Fitness and Sports in Kanoya
- Sachi Ikudome
- Faculty of Physical Education, National Institute of Fitness and Sports in Kanoya
- Hiroki Nakamoto
- Faculty of Physical Education, National Institute of Fitness and Sports in Kanoya
Correspondence: nakamoto@nifs-k.ac.jp
Abstract
Virtual reality is a promising technology that can be used to assess and train decision-making skills in sports. However, it is not necessarily clear what benefits this technology offers compared to previous technologies. The present study investigated the differences in option generation related to tactical decision-making when the same tactical scene was observed in 2D video and 3D VR. Soccer players (N = 27) were asked to observe tactical scenes and verbally report as many tactical options as possible under 2D video and 3D VR conditions. They were classified into high-, medium-, or low-decision capability groups based on the decision-making test. Irrespective of the capability, the 2D video and 3D VR had the same number of generated options. However, 3D VR generated more aggressive options that led to the goal, even though the exact same scene was observed between the conditions. This result may be because 3D VR can reproduce more realistic information exploration behavior than 2D video. Given that differences were observed regardless of skill level, the results suggest that VR technology has the potential for use as a more accurate decision evaluation method and effective training tool compared to previous methods for a wide variety of learners.
1. Introduction
The amount of intensive experience is one of the most important factors in the acquisition of various skills (Ericson et al., 1993; Fitts and Posner, 1967). Therefore, the use of virtual reality (VR), which allows us to experience interaction as if we were present in that physical environment (Kittel et al., 2020), seems to contribute to an increase intensive experience (Appelbaum and Erickson, 2018). For example, sports such as soccer require a large space, many players, and physical resources in practice. Thus, individual daily training is limited to technical skills (e.g., dribbling and shooting) that do not require such resources. On the other hand, it is difficult to practice decision-making (e.g., what play to choose or where to pass), which is the ability to select the best possible option from a variety of alternatives based on the configuration of opponents and their teammates. Given the importance of decision making for success in many complex dynamic sports (Araújo et al., 2006; Williams and Ericsson, 2005; Williams et al., 2011), the above constraints of the practice environment would limit athletes’ skill development. VR has the potential to free players from such constraints, and considering that rich experience facilitates decision-making skills (Miller et al., 2017), it could make a significant contribution. In particular, the emergence of inexpensive stand-alone VR devices that utilize head-mounted displays, such as Meta Quest and VIVE Focus, has rapidly expanded opportunities for individuals to access these environments; several commercial applications are also available that claim to enhance decision-making. However, to understand the effects of VR experience on realistic sports skills, it is important to identify not only the direct training effects of VR (e.g., Fortes et al., 2021) but also what the VR environment specifically brings to the athlete (e.g., Abich et al., 2021; Gray, 2019; Harris et al., 2021; Kittel et al., 2020).
Psychological fidelity has been noted to be particularly important for VR experiences to be beneficial in sports settings (Gray, 2019; Kittel et al., 2020). Psychological fidelity refers to the extent to which a virtual environment recreates the perceptual-cognitive demands of the real task and evokes similar behaviors from the user as in the real environment (Gray, 2019; Miller, 1954). In other words, clarifying what aspects of psychological fidelity VR brings to the user is essential for understanding the effectiveness of the VR experience. Therefore, it is necessary, as a prerequisite, to know the perceptual-cognitive characteristics during decision making that should be reproduced in sports. Decision-making has been studied as a perceptual-cognitive skill in sports science (Willams and Jackson, 2019). The main findings are as follows: in addition to making decisions faster and more accurately than unskilled players (e.g., Diaz et al., 2011; Helsen and Pauwels, 1993; Roca et al., 2011; Stratton et al., 2004; Wein, 2004), skilled players with superior decision-making capability demonstrate more efficient visual search behavior for gathering distributed multiple information such as ball and players on the field (Cañal-Bruland et al., 2011; Mann et al., 2019; North et al., 2009; Roca et al., 2011; Vaeyens et al., 2007a, b; Williams et al., 1994); they can recognize and recall a large amount of multiple information as a meaningful pattern quickly (Didierjean and Marmèche, 2005; van Maarseveen et al., 2015, 2016; Gorman et al., 2013; Ward and Williams 2003); and they can predict the next unfolding events based on the pattern information (North and Williams, 2019). More recently, it has been reported that in decision-making situations, skilled athletes can emerge from a large number of different alternatives that are creative (rare and surprising) or appropriate and useful in a particular situation, based on information obtained through their superior visual search (Roca et al, 2018, 2021a, b). Thus, skilled decision-making is underpinned by the process of gathering and extracting essential information, and by the process of generating multiple promising alternatives and making the best choice among them. In other words, for VR to be a facilitating decision-making experience with high psychological fidelity, it requires an environment that enables information gathering and choice generation, similar to a real situation.
Certain VR increase the psychological fidelity of these processes. In invasive sports, such as soccer and basketball, decisions are made by gathering information spread over a large, 360-degree, depth-rich space. When recreating such scenes, the video images and coaching boards that are frequently used in actual coaching situations are displayed in a scaled-down 2D format, and the player usually observes them from a third-person perspective. In VR, however, a large 360-degree virtual space is represented in 3D and the player observes from a first-person perspective. These differences are expected to have a significant impact on exploratory behavior during information gathering. Several researchers have reported that exploratory behavior, moving the head before receiving a ball, was associated with more successful performance in soccer (Eldridge et al., 2013; Jordet et al., 2013). When a monitor/white board (i.e., 2D video/coaching board) is set up in front of a player, all information on player placement can be perceived with only a slight eye movement. In VR, on the contrary, it is necessary to move the eyes, head, and body in the same way as in a real situation to collect information, thereby limiting the information that can be gathered at the time of gaze orientation. Pagé et al. (2019), who showed the effectiveness of VR-based decision training, suggested that VR visual scenes on head mount display in which head movements are linked to visual scenes, may be more effective than video images because of the greater coupling between participant movement and acquired visual information. In addition, Mann et al. (2009) reported differences in visual search between the first- (i.e., player perspective) and third-person (i.e., aerial perspective) views when soccer players were asked to observe and make decisions. Interestingly, these differences in perspective led to differences in decision making, with the aerial perspective seemingly leading to more accurate decision making (see also, Roca et al., 2018). In other words, the differences in exploratory behavior between 2D video and 3D VR are likely to influence the differences in the gathered information and option generation based on that information. However, it is not clear exactly what kind of difference in decision-making they produce. If the generated options are different, then 3D VR, which offers more realistic exploratory behavior, would be a useful tool to provide a better experience beyond its traditional use in the field (i.e., 2D video/board).
Therefore, this study investigated the differences in option generation for tactical decision-making between a third-person 2D video reproducing tactical scenes typically used in decision-making practice and observing the same tactical scenes in a first-person VR environment. We also examined skill-related differences in this impact. To this end, we first assessed the decision-making ability of individual soccer players using a soccer decision-making test used in decision-making research (Vaeyens et al., 2007a, b) and assigned them to groups. Next, we asked participants to observe a tactical scene presented in 2D video and 3D VR and to generate and verbally report as many options as possible that would be effective in the observed situation. Verbal report protocols help measure the ways in which performers translate information from the visual system into appropriate cognitive processes and behaviors, thus providing deeper insight into the processes that mediate and link perception and tactical decision-making (Roca et al., 2021). A previous study found that better decision-makers have the ability to produce more options (i.e., higher fluency) and generate a greater number of verbal reports of thoughts related to goal scoring (Roca et al., 2018, 2021).
Therefore, in this study, we compared the quantity (number of generated options) and quality (more goal-directed play) of generated options across conditions to investigate the effect of different presentation conditions on decision-making. In the case of 2D, the number of generated options is expected to be large because information is easily obtained, whereas in the case of 3D, decisions with higher priority (options toward the goal) are expected to be made more because players will try to make decisions that are closer to real situations. Furthermore, this tendency is likely to be more pronounced in those with a higher decision-making capacity.
2. Methods
Participants
Twenty-seven male soccer players from university soccer clubs participated in this study. Participants’ playing levels ranged from national-level players who had participated in national tournaments in high school or regularly played in national tournaments at the university level to those who had never played in a national tournament and were currently playing mainly in regional or prefectural leagues. The 27 participants trained for one to two hours per session at least five times a week over a long period of time to improve their competitive performance. All participants were informed of the experimental procedures in advance and consented to participate in the study. This study was approved by the Ethical Review Committee of the administering institution.
Experimental task and apparatus
Decision-making test: First, a decision-making test was conducted to assess the participants’ decision-making ability. The test was based on previous research (Vaeyens et al., 2007a, b). Participants observed 2D video clips of offensive patterns of play near the penalty area and were required to quickly and accurately decide what action the ball carrier should take in that scenario (e.g., to shoot at goal or pass to a particular team mate). The clips showed sequences of play by skilled players who were filmed in each scenario, either acting as defenders or attackers. When filming the clips, players were instructed in which direction they should run, and whether the offensive player should be closely marked by a defender (or not) throughout the clip. All scenarios were filmed from an elevated perspective (approximately 2.3 m above the ground) 3 m behind the center of the field. Defensive and offensive players wore red and white uniforms, respectively. Furthermore, one of the offensive players in the central position wore a yellow uniform. The participants were required to imagine themselves as the player wearing the yellow uniform.
The video clips consisted of attacking scenes that were either 2-versus-1 (that is, 2 attackers [one is a yellow player] versus. One defender), and 3-versus-1, 3-versus-2, 4-versus-3, and 5-versus-3. In addition to the attackers and defenders, a goalkeeper on the defending team was included in all conditions. The players’ positions varied in each situation. Each sequence of play lasted approximately 5 s (range = 3.0–7.3 s), with the clips occluded at the moment when the yellow player received the ball (Vaeyens et al., 2007a, b). The appropriate response (i.e., correct answer) in each situation was determined by the consensus of three soccer coaches licensed by the Japan Football Association who viewed and discussed each clip. Possible responses included passing the ball to a particular teammate (the actual player must be nominated), shooting, or maintaining the possession of the ball. Psychological experimental design software (E-prime 2.0, Psychological Software Tools) was used to create the experimental tasks and present the stimuli. The stimuli were presented on a 65-inch monitor (TH-65PB2J, Panasonic; refresh rate = 60 Hz).
Option generation task: After watching a soccer scene, participants were asked to verbally respond to as many play options as possible in that situation. Participants were presented with a third-person perspective of 2D video or with a first-person perspective of 3D VR scenes of dynamic, 7-versus-7 soccer situations that allowed for a variety of possible options (Figure 1). The presented situations were 20 scenes that were selected as typical decision-making scenes with the consent of one college soccer player and two college soccer coaches who watched 7-versus-7 game scenes. Each stimulus lasted approximately 10 s and was occluded after a 3 s pause at a critical moment (i.e., the moment the participant received a pass from a teammate, a situation in which a variety of tactical options were available). The movements and positions of the players and ball in both stimuli were identical. In the 2D video, the players of the two teams and the ball were presented as figures of different shapes to reproduce magnets like those used on a strategy board (Figure 1A). In 3D VR, the players are represented by triangle columns, and the ball is a soccer ball game object (Figure 1B). In the 2D video condition, one player was circled in red at the beginning of the video, and participants were asked to view the video assuming that the player circled in red was themselves (i.e., they made a decision when the player circled in red received the ball). In the VR condition, the view from the position of the player receiving the ball was presented (i.e., the ball reached the participant at the end).
The positional coordinates of the players used in the stimulus were measured with LPS (ZXY Sports Tracking, Chyronhego, New York, USA) at a sampling frequency of 20 Hz during the 7-versus-7 game mentioned above. A belt with a sensor (approx. 20 g) under their uniform was attached for each player to record the coordinates. The position of the ball was extracted from the video camera images surrounding the pitch using a custom-made MATLAB program (Mathworks ver. 2018b, New York, USA). For the 2D video presentation, a MATLAB program was used to create a movie using the above coordinate data. For the 3D VR presentation, a Unity program (KAIBUTSU lab for soccer, KAIBUTSU, Nagasaki, JAPAN) was used to reproduce the soccer scene by loading the time-series data of the coordinates of the players and the ball. The virtual environment consisted of a soccer stadium with a soccer field and regular-sized goal.
Stimuli were presented on a 65-inch monitor (TH-65PB2J, Panasonic; refresh rate = 60 Hz) in the 2D video condition. In the 3D VR condition, an HTC Vive head-mounted display VR system (HTC Corporation, New Taipei City, Taiwan) was used for the experiment. The system consisted of a headset and two lighthouses that emit infrared laser sweeps to localize the headset. HTC Vive has excellent spatial and temporal precision (Borrego et al., 2018; Niehorster et al., 2017). Participants wore the Vive headset to view the virtual environment and attempted to verbalize the options they came up with immediately after occlusion of the scene.
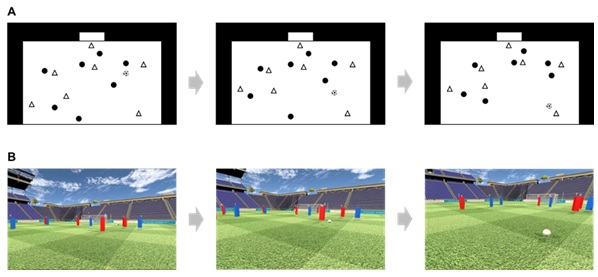
Procedure
Decision-making test: Participants performed the decision-making test while standing in front of a monitor. We asked participants to make their decisions as quickly and accurately as possible once the ball was played in the direction of the yellow player. We asked participants to respond with a single button press at the moment they decided their action to measure the time taken to make a decision. We occluded the film sequence immediately after the button press to prevent participants from changing their response after receiving additional information after the button press, but before verbally reporting their decision.
The participants first performed five practice trials to familiarize themselves with the experimental setting. Next, we presented 34 offensive patterns: three 2-versus-1, ten 3-versus-1, six 3-versus-2, six 4-versus-3, and nine 5-versus-3 situations, following previous studies (Vaeyens, 2007a, b). The order of the video clips was randomized. The interval between trials was approximately 30 s, and participants completed the entire test session in approximately 30 min.
Option generation task: Participants performed the option generation task after fitting the VR head-mounted display. We asked participants to verbally report as many play options as possible in that situation after receiving the pass from their teammates in the VR space. Participants first performed six practice trials for familiarization with the virtual environment. Next, we presented 20 soccer scenes. The order of the presented scenes was randomized. The interval between trials was approximately 10 s, and participants completed the entire test session in approximately 30 min.
Dependent variables and data analysis
First, decision time and correctness were evaluated in each trial of the decision test to classify the participants into groups based on the results of the decision test. For the decision time evaluation, we ranked the decision time of all participants in each trial, and the average ranking of all 34 trials was used as the participant’s score. For correctness, each participant was ranked based on the percentage of correct responses, and this ranking was used as the participant’s score. These two scores were summed and the participants were classified into three groups (high-, medium-, or low-decision capability), with nine participants in each group.
Next, we evaluated the number of play patterns generated by the participants and the quality of play in both the 2D video and 3D VR conditions. To evaluate the quality of play, first, two coaches with a Japan Football Association coaching license evaluated whether the play patterns reported by the participants were options that directly led to the goals. It has been reported that soccer is a game of thinking about the situation in front (attacking direction) and moving forward, and that reducing passes to the side and back and advancing the ball forward leads to scoring opportunities (Bate, 1988). Based on this, in this study, two coaches separately scored each play reported by the participants as follows: 2 points for a play that could lead to a goal or an assist, 1 point for a play that could change the situation, and 0 points for a play that was less likely to change the situation. The correlation between the scores of the two instructors for each trial was r = 0.87 (2D video) and r = 0.92 (3D VR) (Figure 2). From these results, it can be concluded that the evaluation criteria for the two coaches were almost identical. Therefore, we evaluated the quality of play by taking the average of the two coaches as the score and dividing it by the number of generated play patterns. In other words, the higher the score, the higher the quality of the play, which directly leads to goal scoring. The number of option generations and quality score data were subjected to a 3 (group: high-, medium-, or low-decision capability group) × 2 (stimulus presentation type:2D video and 3D VR) ANOVA. Degrees of freedom for F-ratios were adjusted using the Greenhouse–Geisser procedure if violations to sphericity were encountered. All post-hoc pairwise comparisons were performed using the Bonferroni test. Effect sizes were estimated using the partial eta-squared measure (ηp2). Statistics were conducted using IBM SPSS Statistics 25. The significance level was set at 5%.
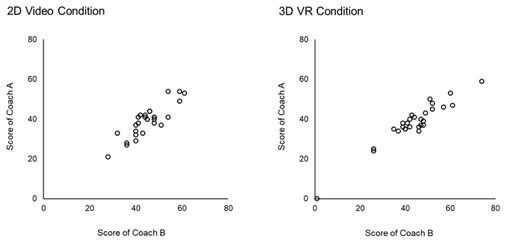
for the two coaches
3. Results
Analysis of variance for the number of option generations (Figure 3A) showed no significant interaction (F (2, 24) = 0.83, p > .05, ηp2 = .06) and no significant main effects of group (F (2, 24) = 0.32, p > .05, ηp2 = .03) or stimulus presentation condition (F (1, 24) = 1.85, p > .05, ηp2 = .07). In other words, the type of stimulus presentation did not produce quantitative differences in option generation. This result was similar for all groups with different decision-making abilities.
Next, an analysis of the option quality score (Figure 3B), which reflects the quality of the generated options, showed that while there was no main effect of group (F (2, 24) = 0.16, p > .05, ηp2 = .01), there was a significant main effect of stimulus presentation type (F (1, 24) = 9.86, p < .01, ηp2 = .29). In other words, the results indicated higher scores for 3D VR videos than for 2D videos.
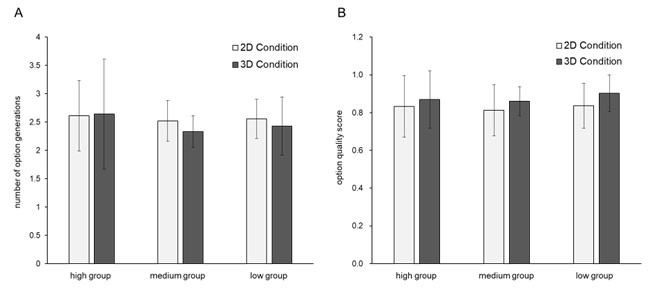
for 2D and 3D conditions in each group
4. Discussion
This study investigated the differences in option generation for tactical decision-making between the types of stimulus presentation (i.e., third-person 2D video and a first-person VR environment). We also examined whether the impact of these differences on decision-making varies with the level of decision-making ability. No significant difference was found in the number of generated options in the decision-making situation between the stimulus presentation types, but in terms of the quality of the options, regardless of the skill level, it was found that the participants generated more goal-scoring directed options in the 3D VR. These results suggest that 3D VR may be a useful tool for inducing the exact perceptual-cognitive processes (i.e., higher psychological fidelity) involved in decision-making.
The most striking finding was that the generated options differed depending on the type of stimulus presentation, even though the participants observed the same exact tactical situation. In this study, based on the idea that soccer is a game in which fewer passes to the side or back and more forward movement of the ball lead to scoring chances (Bate, 1988), we assigned higher scores to participants’ verbal responses when they were judged to lead to goal scoring. As a result, regardless of skill level, the participants demonstrated higher scores in the 3D VR than in the 2D video. That is, they generated more goal-scoring directed options in 3D VR.
One reason for this may be the high psychological fidelity of exploratory behaviors in 3D VR. It has been shown that information exploration behavior varies with the number of players (Vaeyens et al., 2007a), perspectives (Mann et al., 2009), or dimensions (Put et al., 2014) of the presented stimuli and methods, and differences in gaze behavior and differences in the content of the collected information have been reported to influence decision making (Mann et al., 2009; Roca et al., 2018). As described in the introduction, 3D VR in this study is characterized by the fact that information is gathered by moving the eyes, head, and body, unlike in 2D video. Owing to this feature, all information on the pitch can be easily obtained in a short time in 2D video, whereas in 3D VR, the load of information collection is higher and the same amount of information cannot be obtained in the same amount of time. Such temporal constraints are thought to alter gaze behavior (e.g., Navia et al., 2017). Furthermore, Musclus et al. (2021) reported that the presence of time constraints leads to the generation of fewer but higher-quality decision options than the absence of time constraints (see also, Musclus, 2018). Johnson and Raab (2003) proposed the Take-the-First heuristic strategy in decision-making (see also, Raab, 2012). Accordingly, players generate options in the order of validity (search), stop after two to three options (stop), and select one of the initial options generated (decision). Considering the above, it is possible that due to the high load (time constraints) of exploratory behavior in 3D VR, the participants could be focused on high-priority information (information to lead them to the goal), and as a result, more attacking options might be preferentially reported. In contrast, additional information than usual, such as 2D video, may have resulted in different tactical decision-making options being reported preferentially than in the actual situation. Furthermore, the heuristics view seems to fully explain the lack of difference in the number of generated options between conditions in the present study (the number of reported options were actually around 2-3). Importantly, in real-world decision-making under time constraints, not all pitch information is necessarily available during play. Therefore, 3D VR, which induces such exploratory behavior, is beneficial because of its high psychological fidelity to the decision-making process. However, this study did not examine specific changes in information gathering due to differences in exploratory behavior. In the future, the causes of differences in decision making will become clearer if the measurement of gaze behavior is included.
It is also possible that the characteristics of VR’s high similarity and representativeness of visual information in real-life situations also had an impact. The necessity of including visual information similar to real-world environments has been emphasized for the assessment and training of perceptual-cognitive skills (Brunswik, 1956; Hadlow et al., 2018; Pinder et al., 2011; Travassos et al., 2013) because the stimuli become more similar to the real environment, the advantage of skilled players over novices becomes more pronounced (Mori et al., 2002; Shim et al., 2005; Lynch et al., 2019) and, the transferability of the training effect to real environments becomes higher (Hadlow et al., 2018). This is because the perceptual-cognitive skills of skilled players are not based on basic abilities (e.g., simple reaction time or visual acuity) but on domain-specific knowledge (e.g., play patterns) developed through past experiences (Williams et al., 1999). In other words, visual information must closely recreate the essential information that induces this knowledge (e.g., the placement of the players in the game). Cognitive psychology research has shown that recall is superior when the environment is the same as that in which learning takes place (Godden and Baddeley 1975). According to this notion, the immersive feeling of being in the actual playing environment (e.g., stadium and/or soccer field) provided by VR seems to be an important factor that influences memory recall. In other words, soccer players who make decisions based on their memories of past pattern information may have had context-dependent memories evoked in the VR environment, preferentially guiding their goal-directed decisions used in actual situations.
Thus, the decision options recalled on 2D video/coaching boards, which are frequently used in decision training in real-life situations, appear to differ from those generated in 3D VR. These results suggest that VR has high psychological fidelity beyond previous technologies and could be used for more effective decision evaluation and training. Common practice involves pausing play and allowing the instructor to correct the players’ decisions. This method requires a lot of resources and can only be performed in a limited amount of time. To compensate for this limitation, the use of 3D VR has certain advantages. In particular, it may contribute to improving the ability to generate options that are directly related to the goal. Thus, the finding that VR training is more effective than video image training in terms of transitions (Fortes et al., 2021; Pagé et al., 2019) may be because VR involves more realistic and flexible decision-making processes. In addition, we initially thought that these effects of 3D VR would be more pronounced for those with higher skill levels, but this was not the case. In other words, the scope of VR use is not limited to the skill level but could be effective at any level. However, a more detailed examination of gaze measurement and decision-making processes, as well as controlled training experiments, are needed to confirm these findings.
Author Contributions
Conceptualization, S.Y., D.M., and H.N.; methodology, S.Y. and D.M., and H.N.; software, H.N. and Y.T.; validation, D.M., S.I. and H.N.; formal analysis, D.M.; investigation, S.Y.; data curation, S.Y.; writing—original draft preparation, D.M.; writing—review and editing, H.N.; visualization, S.I.; project administration, H.N.; funding acquisition, H.N,, and D.M.
Funding
This research was supported by grants-in-aid for scientific research from the JSPS (No. 19H04001) to HN and the JSPS (No. 22K17740) to DM.
Institutional Review Board Statement
The study was conducted according to the Declaration of Helsinki, and approved by the Institutional Review Board of the National Institute of Fitness and Sports in Kanoya.
Informed Consent Statement
Informed consent was obtained from all participants involved in the study.
Conflicts of Interest
The authors declare no conflict of interest.
References
Abich, J. et al. (2021). A review of the evidence for training effectiveness with virtual reality technology, Virtual Reality, 25(4), 919–933. https://doi.org/10.1007/s10055-020-00498-8
Appelbaum, L. G., & Erickson, G. (2018). Sports vision training: A review of the state-of-the-art in digital training techniques, International Review of Sport and Exercise Psychology, 11(1), 160–189. https://doi.org/10.1080/1750984X.2016.1266376
Araújo, D., Davids, K., & Hristovski, R. (2006). The ecological dynamics of decision making in sport, Psychology of Sport and Exercise, 7 (6), 653–676. https://doi.org/10.1016/j.psychsport.2006.07.002
Bate, R. (1988). Football chance: Tactics and strategy. In T. Reilly, A. Lees, K. Davids and W. Murphy (Eds.). Science and Football. E. & F.N. Spon, London, pp. 293–301.
Borrego, A. et al. (2018). Comparison of Oculus Rift and HTC Vive: Feasibility for Virtual Reality-Based Exploration, Navigation, Exergaming, and Rehabilitation, Games for Hearth Journal, 7(3), 151–156. https://doi.org/151-156. 10.1089/g4h.2017.0114
Brunswik, E. (1956). Perception and the representative design of psychological experiments (2nd ed.). Berkeley: University of California Press.
Cañal-Bruland, R. et al. (2011). Visual span and change detection in soccer: An expertise study, Journal of Cognitive Psychology, 23(3), 302–310. https://doi.org/10.1080/20445911.2011.496723
Diaz del Campo, D. G. et al. (2011). Differences in decision-making development between expert and novice invasion game players, Perceptual and motor skills, 112(3), 871–888. https://doi.org/10.2466/05.10.11.25.PMS.112.3.871-888
Didierjean, A. & Marmèche, E. (2005). Anticipatory representation of visual basketball scenes by novice and expert players, Visual Cognition, 7(2), 149–158. https://doi.org/10.1080/13506280444000021A
Diederick, C., Li Li, & Lappe, M. (2017). The Accuracy and Precision of Position and Orientation Tracking in the HTC Vive Virtual Reality System for Scientific Research, Iperception, 8(3): 2041669517708205. https://doi.org/10.1177/2041669517708205
Eldridge, D., Pulling, C., & Robins, M. T. (2013). Visual exploratory activity and resultant behavioural analysis of youth midfield soccer players, Journal of Human Sport and Exercise, 8(3), 560–577. https://doi.org/10.4100/jhse.2013.8.Proc3.02
Ericsson, K. A., Krampe, R. T., & Tesch-Römer, C. (1993). The role of deliberate practice in the acquisition of expert performance, Psychological Review, 100(3), 363–406. https://doi.org/10.1037/0033-295X.100.3.363
Fitts, P. M., & Posner, M. I. (1967). Human performance, p.162. Oxford, England: Brooks/Cole.
Fortes, L. et al. (2021). Virtual reality promotes greater improvements than video-stimulation screen on perceptual-cognitive skills in young soccer athletes, Human Movement Science, 79: 102856. https://doi.org/10.1016/j.humov.2021.102856
Godden, D.R., & Baddeley, A. D. (1975). Context-dependent memory in two natural environments: on land and underwater, British Journal of Psychology, 66(3),325–331. https://doi.org/10.1111/j.2044-8295.1975.tb01468.x
Gorman, A. D., Abernethy, B., & Farrow, D. (2013). Is the relationship between pattern recall and decision-making influenced by anticipatory recall? Quarterly Journal of Experimental Psychology, 66(11), 2219–2236. https://doi.org/10.1080/17470218.2013.777083
Gray, R. (2019). Virtual environments and their role in developing perceptual-cognitive skills in sports, In: Williams AM, Jackson RC (eds) Anticipation and Decision Making in Sport. Taylor and Francis, Routledge.
Hadlow, S. M. et al. (2018). Modified perceptual training in sport: A new classification framework, Journal of Science and Medicine in Sport, 21(9), 950–958. https://doi.org/10.1016/j.jsams.2018.01.011
Harris, D. J. et al. (2021). Assessing the learning and transfer of gaze behaviours in immersive virtual reality, Virtual Reality, 25, 961–973. https://doi.org/10.1007/s10055-021-00501-w
Helsen, W., & Pauwels, J. M. (1993). The relationship between expertise and visual information processing in sport, In J. L. Starkes & F. Allard (Eds.), Cognitive issues in motor expertise, 109–134.
Johnson, J. G., & Raab, M. (2003). Take the first: Option-generation and resulting choices, Organizational Behavior and Human Decision Processes, 91(2), 215–229. https://doi.org/10.1016/S0749-5978(03)00027-X
Jordet, G., Bloomfield, J., & Heijmerikx, J. (2013). The hidden foundation of field vision in english premier league (EPL) soccer players, MIT Sloan Sports Analytics Conference, Boston, MA.
Kittel, A. et al. (2020). Effectiveness of 360°Virtual Reality and Match Broadcast Video to Improve Decision-making Skill, Science and Medicine in Football, 4(6), 255–262. https://doi.org/10.1080/24733938.2020.1754449
Lynch, S. D. et al. (2019). Detection of deceptive motions in rugby from visual motion cues, PLoS One, 14(9), e0220878. https://doi.org/10.1371/journal.pone.0220878
Mann, D. L. et al. (2009). The influence of viewing perspective on decision-making and visual search behaviour in an invasive sport, International Journal of Sport Psychology, 40(4), 546–564. https://doi.org/10.1016/j.humov.2008.08.003
Mann, D. L. et al. (2019). Visual search behaviours in expert perceptual judgements. In A. M. Williams & R. C. Jackson (Eds.), Anticipation and decision making in sport (pp. 59–78) .Routledge/Taylor & Francis Group.
Miller, A. et al. (2017). Exposing athletes to playing form activity: outcomes of a randomised control trial among community netball teams using a game-centred approach, Journal of Sports Sciences, 35(18), 1–12. https://doi.org/10.1080/02640414.2016.1240371
Miller, R. (1954). Psychological considerations in the design of training equipment, Report no. WADC-TR-54-563, AD 71202, Wright Patterson Air Force BAse, OH; Wright Air Development Center. Wright-Patterson Air Force Base.
Mori, S., Ohtani, Y., & Imanaka, K. (2002). Reaction times and anticipatory skills of karate athletes, Human Movement Science, 21(2), 213–230. https://doi.org/10.1016/s0167-9457(02)00103-3
Musculus, L. (2018). Do the best players “take-the-first”? Examining expertise differences in the option-generation and selection processes of young soccer players, Sport, Exercise, and Performance Psychology, 7(3), 271–283. https://doi.org/10.1037/spy0000123
Musculus, L. et al. (2021). The Influence of Environmental Constraints in 360°Videos on Decision Making in Soccer, Journal of sport & exercise psychology, 43(5), 365–374. https://doi.org/10.1123/jsep.2020-0166
Navia, J. A. et al. (2017). Gaze control during interceptive actions with different spatiotemporal demands. Journal of experimental psychology, Human perception and performance, 43(4), 783–793. https://doi.org/10.1037/xhp0000347
North, J. S., & Williams, A. M. (2019). Familiarity detection and pattern perception. In A. M. Williams & R. C. Jackson (Eds.), Anticipation and decision making in sport. Abingdon, UK: Routledge. 25–42.
North, J. S. et al. (2009). Perceiving patterns in dynamic action sequences: underpinning stimulus recognition and anticipation skill, Cognitive Psychology, 23, 878–894. https://doi.org/10.1002/acp.1581
Pagé C., Bernier P. M., & Trempe, M. (2019). Using video simulations and virtual reality to improve decision-making skills in basketball, Journal of Sports Sciences, 37(21), 2403–2410. https://doi.org/10.1080/02640414.2019.1638193
Pinder, R. A. et al. (2011). Representative learning design and functionality of research and practice in sport, Journal of Sport & Exercise Psychology, 33 (1), 146–155. https://doi.org/10.1123/jsep.33.1.146
Put, K. et al. (2014). The use of 2D and 3D information in a perceptual-cognitive judgement task, Journal of sports sciences, 32(18), 1688–1697. https://doi.org/10.1080/02640414.2014.912760
Raab, M. (2012). Simple heuristics in sports, International Review of Sport and Exercise Psychology, 5(2), 104–120. https://doi.org/10.1080/1750984X.2012.654810
Roca, A., & Ford, P.R. (2021a). Developmental activities in the acquisition of creativity in soccer players, Thinking Skills and Creativity, 41, 100850. https://doi.org/10.1016/j.tsc.2021.100850
Roca, A., Ford, P. R., & Memmert, D. (2018). Creative decision making and visual search behavior in skilled soccer players, PLoS ONE, 13(7), e0199381. https://doi.org/10.1371/journal.pone.0199381
Roca, A., Ford, P.R., & Memmert, D. (2021b). Perceptual-cognitive processes underlying creative expert performance in soccer, Psychological Research, 85, 1146–1155. https://doi.org/10.1007/s00426-020-01320-5
Roca, A. et al. (2011). Identifying the processes underpinning anticipation and decision-making in a dynamic time-constrained task, Cognitive processing, 12(3), 301–310. https://doi.org/10.1007/s10339-011-0392-1
Shim, J. et al. (2005). The Use of Anticipatory Visual Cues by Highly Skilled Tennis Players, Journal of Motor Behavior, 37 (2), 164–175. https://doi.org/10.3200/JMBR.37.2.164-175
Stratton, G. et al. (2004). Youth Soccer: From Science to Performance, Routledge, London.
Travassos, B. et al. (2013). Performance analysis in team sports: Advances from an Ecological Dynamics approach, International Journal of Performance Analysis in Sport, 13, 83–95. https://doi.org/10.1080/24748668.2013.11868633
Vaeyens, R. et al. (2007a). The effects of task constraints on visual search behavior and decision-making skill in youth soccer players, Journal of Sports & Exercise Psychology, 29(2), 147–169. https://doi.org/10.1123/jsep.29.2.147
Vaeyens, R. et al. (2007b). Mechanisms underpinning successful decision making in skilled youth soccer players: an analysis of visual search behaviors, Journal of motor behavior, 39(5), 395–408. https://doi.org/10.3200/JMBR.39.5.395-408
van Maarseveen, M. J., Oudejans, R. R. D., & Savelsbergh, G. J. P. (2015). Pattern recall skills of talented soccer players: Two new methods applied, Human Movement Science, 41, 59–75. https://doi.org/10.1016/j.humov.2015.02.007
van Maarseveen, M. J. et al. (2016). Perceptual-cognitive skill and the in situ performance of soccer players, Quarterly Journal of Experimental Psychology, 71(2), 1–17. https://doi.org/10.1080/17470218.2016.1255236
Ward, P. & Williams, A. M. (2003). Perceptual and Cognitive Skill Development in Soccer: The Multidimensional Nature of Expert Performance, Journal of Sport and Exercise Psychology, 25(1),93–111. https://doi.org/10.1123/jsep.25.1.93
Wein, H. (2004). Developing Game Intelligence in Soccer. Reedswain, Spring City.
Williams, A. M. et al. (1994). Visual search strategies of experienced and inexperienced soccer players, Research Quarterly for Exercise and Sport, 65(2), 127–135. https://doi.org/10.1080/02701367.1994.10607607
Williams, A. M., & Ericsson, K. A. (2005). Perceptual-cognitive expertise in sport: Some considerations when applying the expert performance approach, Human Movement Sciences, 24, 283–307. https://doi.org/10.1016/j.humov.2005.06.002
Willams, A. M., & Jackson, R. C (2019). Familiarity detection and patter perception, In A. M. Williams & R. C. Jackson (Eds.), Anticipation and decision making in sport (pp. 25–42), Routledge/Taylor & Francis Group.
Williams, A.M., Davids, K., & Williams, J. G. (1999). Anticipation and decision-making in sport, In A. M. Williams, Davids, K., & Williams, J. G. (Eds.), Visual Perception and action in Sport (pp. 97–144), Routledge/Taylor & Francis Group.
Williams, A. M. et al. (2011). Perceptual-cognitive expertise in sport and its acquisition: implications for applied cognitive psychology, Applied Cognitive Psychology, 25, 432–442. https://doi.org/10.1002/acp.1710
Relevant Articles
-
An Inquiry-Based Learning Support System for Children in Sports Acquisition Processes
by Masayuki Yamada - 2024,3
VIEW -
Clarifying the Sharpened network diversity in French flair rugby
by Koh Sasaki - 2024,2
VIEW -
Review matching task to diagnose basic review ability
by Koki Saito - 2024,1
VIEW -
Japanese elementary teachers’ problem-based learning through online professional development on teaching Japanese language learners in physical education
by Takahiro Sato - 2024,S1
VIEW